In my previous article Forward Price Uncertainty in Renewable Markets and its Effect on Offtake Contracts, I explored how loss of price elasticity and price-risk adjustments in Power Purchase Agreements (PPAs) and energy hedges contribute to declining liquidity in renewable energy markets. These challenges stem from the difficulty of aligning future supply with future demand, particularly for Independent Power Producers (IPPs) / Renewable Capacity Providers (RCPs), who face significant risks. Factors like the variability of renewable energy production and fluctuating demand from electric vehicles, AI data centers, EV charging stations, blockchain mining, and energy storage amplify the difficulty of achieving Renewable Portfolio Standards (RPS) of roughly 30% or more. However, this only partially explains the issue.
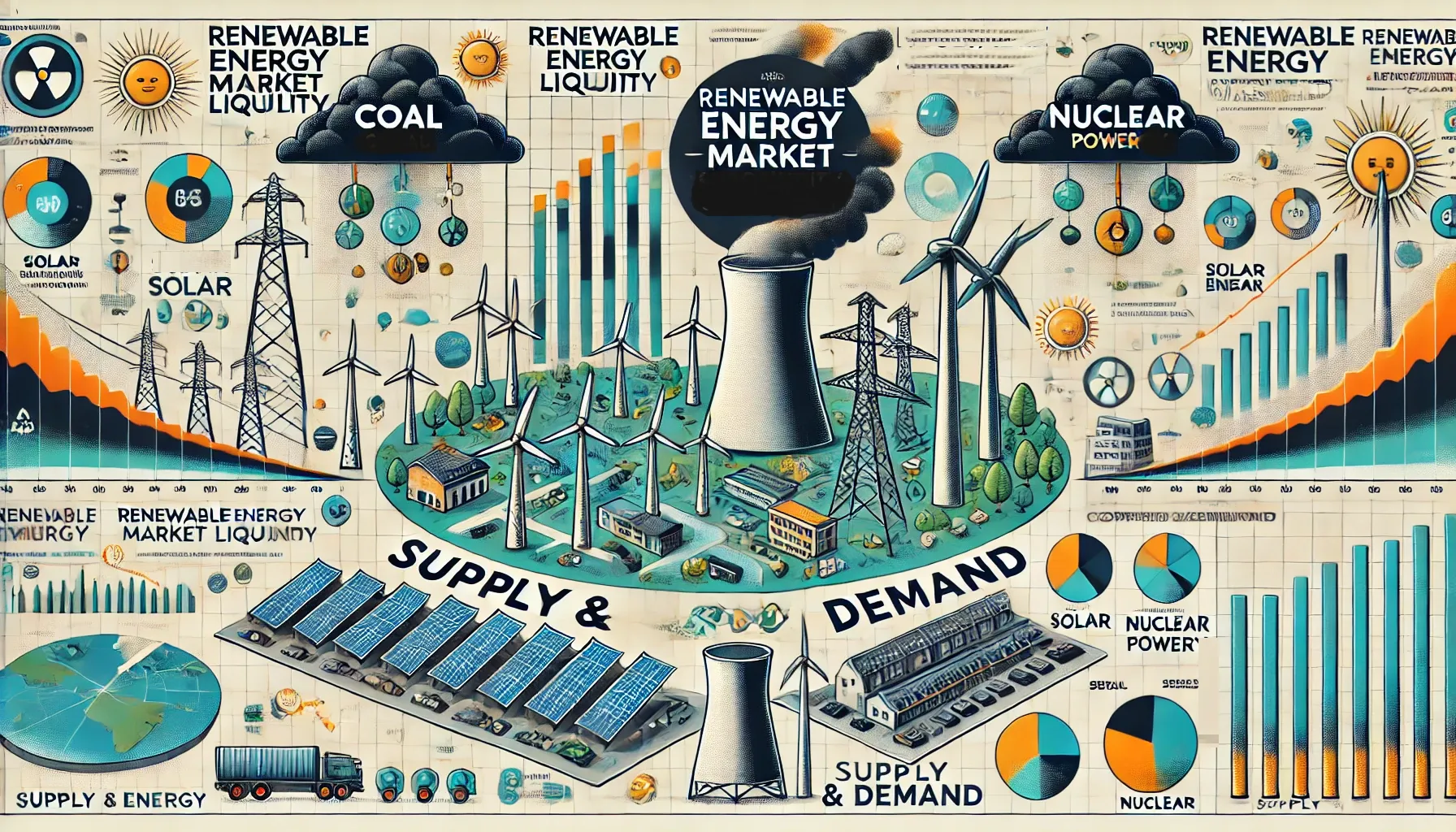
The Impact of Tranching and Price Fragmentation
The lack of market liquidity in renewable energy markets is largely due to tranching where the market distinguishes baseline load from peak load, and price fragmentation where prices are unevenly distributed across a hub which arises from Locational Marginal Pricing (LMP) based on economic dispatch. These models and market structures were originally designed for centralized, dispatchable energy sources (e.g., coal, nuclear, hydro) to match predictable baseline and intermediate demand. However, they create challenges for handling the variability and sudden surges of renewable energy. This structural misalignment has prevented the development of an integrated futures market for renewable energy, disrupting liquidity in both long-term offtake contracts and short-term energy trading. Viable solutions are therefore limited.
Historic Market Design — Tranching
Historically, electricity markets were designed to balance predictable baseline demand using dispatchable resources like natural gas turbines for minor, short-term adjustments. This approach was sufficient when both demand variability and peak-load fluctuations were relatively limited. Ancillary services such as spinning and non-spinning reserves, designed to provide quick-response capacity, were typically maintained at just 1–3% of baseline load. During this era, demand closely followed predictable patterns, enabling seasonal forecasts to align well with actual baseline load. As a result, real-time adjustments were primarily needed for peak-demand deviations, which could be addressed through dispatchable energy in intermediate and short-term markets.
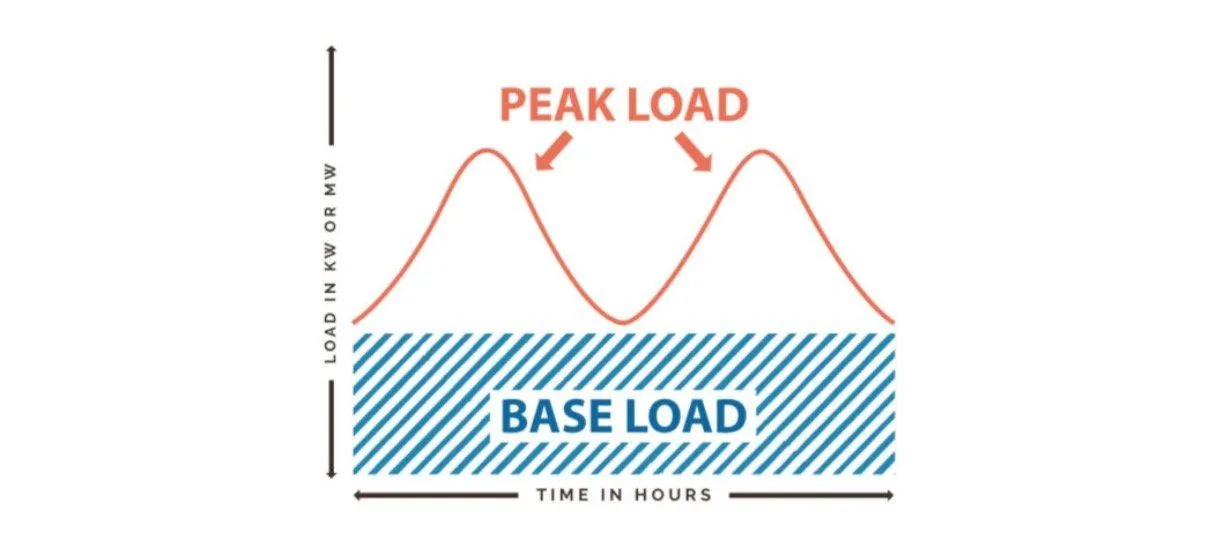
Market structures evolved to reflect this predictability, with baseline load largely served by forward power markets and short-term deviations managed via Day-Ahead and Real-Time Markets. These markets, covering intervals from 48 hours to one hour before delivery, ensured sufficient flexibility without requiring significant liquidity. Ancillary reserves were located near industrial demand centers to respond quickly to deviations between real-time demand and supply.
However, the rise of renewable energy has introduced significant challenges. The variability and uncertainty of non-dispatchable resources such as wind and solar have increased the need for ancillary services and market coordination. Operators now aim to shift peak demand to align with renewable supply forecasts, a strategy that requires enhanced “just-in-time” coordination and greater reliance on ancillary reserves. Unlike dispatchable resources, renewable generation often produces energy far from demand centers, creating mismatches that cannot easily be resolved without storage or transmission upgrades. Excess renewable generation is frequently curtailed when local demand cannot absorb it, leading to inefficiencies.
This shift has exposed limitations in traditional market structures. Short-term markets, which historically required limited liquidity due to smaller reserve needs, now face increased pressure. The relative illiquidity of these markets compared to forward markets stems from their smaller share of total energy transactions. Furthermore, the financial mechanisms supporting short-term markets remain underdeveloped relative to the growing need for flexibility and responsiveness, especially as renewable energy continues to transform the supply landscape
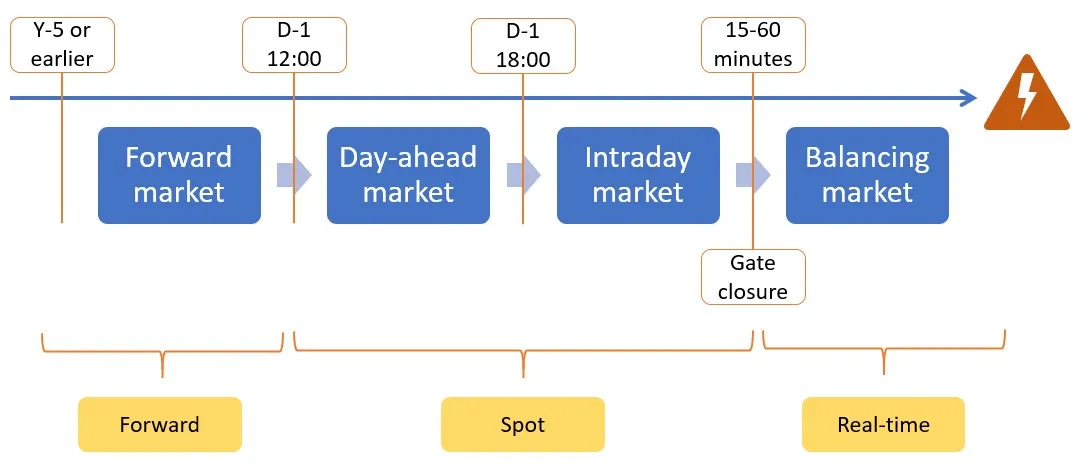
Modern energy trading, apart from capacity and transmission rights markets, relies heavily on segmenting energy procurement (i.e. tranching) to balance deviations from baseline and mid-merit load. However, frequent and periodic peak-load variance relative to supply — particularly during periods of renewable overgeneration — challenges this framework. When significantly more energy is produced than baseline demand can accommodate, the traditional supply-demand balancing mechanisms face increased strain. This mismatch introduces additional uncertainty into market operations and requires enhanced flexibility in ancillary services, storage solutions, and real-time market coordination.
Why we can’t we upgrade the grid and market infrastructure to accommodate peak-load variance?
The first reason is that renewable energy output cannot exceed the grid’s capacity to absorb it in conjunction with non-renewable baseline supply (coal, nuclear, and hydro). For example, baseline load is typically supplied by non-renewable resources, which would need to ramp down rapidly during large-scale renewable energy surges to make room for non-dispatchable energy. However, ramping down traditional baseload generators like coal and nuclear within a short timeframe (e.g., under 24 hours) may not be feasible due to their slower response times and/or existing contractual obligations. While hydro can offer more flexibility, it is often limited by geographic and seasonal factors.
Additionally, only a small portion of renewable energy can reliably be integrated as baseline or mid-merit load under the current market structure. This portion, often represented as the P90 (90th percentile probability of exceedance), reflects the energy output that can be counted on with high reliability. For wind farms, this value is often far less than 50% of their nameplate capacity, leaving a significant amount of excess energy that current short-term markets and grid infrastructure are ill-equipped to handle. The unutilized capacity frequently results in curtailment or inefficiencies, as the short-term markets were not designed to absorb rapid and unpredictable renewable surges.

The bad news — As more wind and solar farms are added, the challenges associated with renewable integration worsen. Additional peak supply is often pushed into illiquid short-term markets, leading to curtailment when equivalent demand cannot be shifted within the available time window. Under current market structures, achieving high Renewable Portfolio Standards (RPS) of 30% or more could become increasingly difficult without significant changes to grid infrastructure and market mechanisms.
The good news — It is possible to increase the baseline utilization of renewables through aggregate tranching, which pools renewable resources to provide a more reliable baseline energy supply. By coordinating this process using improved protocols, advanced forecasting techniques, and system-wide heuristics, the overall reliability of renewable energy can increase as a percentage of total capacity. This requires a well-coordinated ecosystem of Renewable Capacity Providers (RCPs). (For more details, see our white paper or future articles.)
Grid upgrades present another major challenge. Modifying the grid is both economically and politically sensitive, requiring careful planning over a long timeframe. A shift from a baseline-load-focused market to one that prioritizes on-demand flexibility would require massive investments in transmission capacity, spinning and non-spinning reserves, and other ancillary services. Such a transition carries risks, including grid instability issues like Area Control Error (ACE), brownouts, or worse.
Furthermore, existing ancillary and reserve markets are still largely dominated by traditional resources like natural gas turbines. Increasing the capacity of these assets to accommodate renewable variability can conflict with Renewable Portfolio Standards (RPS), as it reduces the relative share of renewable energy. However, advancements in storage technologies and renewable-compatible ancillary services may provide a path forward.
Historic Market Design — Fragmentation
Another form of market disaggregation worth noting is what we refer to as market or price fragmentation — i.e., the division of energy markets into distinct price nodes to reflect supply-demand variance at different points of interconnection, a mechanism commonly known as Locational Marginal Pricing (LMP). LMP accounts for localized factors such as transmission constraints, line losses, equipment inefficiencies (e.g., transformers, inverters), and powerline repairs. These factors create supply-demand imbalances across nodes, resulting in price disparities even within a given hub or region.
This localized price variability has significant implications for energy producers, particularly Independent Power Producers (IPPs) with variable-output assets like wind or solar farms. The price paid to these producers is determined by the node where they interconnect, and fluctuations at the node can disrupt cash flows. To mitigate this volatility, IPPs often rely on price swaps or offtake contracts to stabilize revenues — i.e. PPA or energy hedge. (For more details on offtake contracts, see my previous article )
The integration of variable renewable energy, such as wind and solar, introduces additional complexities. During periods of high renewable output, peak supply often exceeds baseline, intermediate, and even peak demand. Because renewable energy must be absorbed quickly and because markets are most illiquid during these periods, curtailments frequently occur. These curtailments reduce the effective output of renewable assets, often leaving them operating well below their full capacity to maintain grid stability and balance supply and demand.
Tranching and Fragmentation Create Illiquid Renewable Markets
The combination of market tranching and fragmentation significantly contributes to the illiquidity of renewable energy markets. Here’s how these factors interact:
1) Market Tranching and Risk Management
Market tranching, often employed in offtake contracts, introduces price and shape risk deductions under Power Purchase Agreements (PPAs) or baseline volume deductions in Energy Hedges. This conservative approach arises from the inherent difficulty in predicting how much renewable energy will be forced into short-term, illiquid markets. Non-renewable assets supplying firm baseline load further constrain the ability to hedge renewables reliably in forward and intermediate markets. As a result, only a fraction of renewable capacity is typically hedged, leaving much of it exposed to short-term volatility.
2) Market Fragmentation and Transmission Constraints
Market fragmentation, driven by Locational Marginal Pricing (LMP), exacerbates illiquidity in short-term markets. Renewable energy often ramps up in regions far from demand centers where transmission infrastructure may be insufficient to transport surplus energy. Short-term markets, traditionally reliant on fast-dispatchable resources like natural gas turbines near demand centers, are ill-equipped to absorb large, sudden increases in renewable supply. Consequently, curtailments become the default solution, prioritizing grid stability over renewable integration.
3) Challenges with Combining PPAs and Hedges
Combining PPAs and Energy Hedges for renewable assets is complex due to variability in output and the difficulty of aggregating contracts between multiple producers and demand-side market participants. Individual producers often operate independently, leading to suboptimal market outcomes. Additionally, these contracts typically cover only the P90 production level (90th percentile probability of exceedance), which often represents less than 50% of total capacity, leaving a substantial portion unhedged and subject to illiquidity.
4) Opportunities in Geographical Diversification
Despite these challenges, a geographically dispersed portfolio of renewable assets can improve overall baseline capacity utilization. Variations in production profiles across regions often offset each other, leading to greater aggregate production certainty as a percentage of installed capacity. However, achieving this requires significant coordination between asset owners, who frequently view one another as competitors. Addressing this lack of cooperation is critical to unlocking the potential of renewables in serving baseline demand.
The Challenge of Storage Solutions
While advanced battery systems could theoretically smooth peak-load variance, serious practical challenges remain. The two primary issues are listed below:
- Economic Viability: Batteries require a predictable price differential between charging (low prices) and discharging (high prices). However, peak price forecasting remains inaccurate, making optimal battery scheduling difficult. Note that prices drop whenever renewable energy increases in aggregate but they don’t necessarily peak when renewable energy dissipates — peak price forecasting is thus like looking for a needle in a haystack. The discharge window is also likely to be short due to the inaccuracy of peak-price forecasts until very close to the peak pricing event — discharging outside this window may not be economically viable.
- Performance Limitations: Frequent cycling, especially when the battery is fully discharged, damages battery systems, and slower ramp rates reduce their effectiveness compared to traditional spinning reserves. This precludes the use of battery storage systems to act as spinning reserves for instance. As a result, storage solutions are often insufficient to address short-term liquidity gaps — i.e. ramp up (+) or ramp down (-).
Consequently, its quite challenging for batteries to optimally time between charge and discharge periods although some improvements have been made in this area as of late and it is currently an ongoing area of research.
Why a Market-Based Solution is Needed
A recent article from Wood Mckinsey emphasizes the need to adopt a market-based solution given that the market and infrastructure are both designed to serve baseline load with dispatchable energy vs peak load using non-dispatchable energy.
“The current electricity grid market design may need a fundamental shift to deliver a successful and orderly energy transition. The current market design is optimized for baseload production — thus heavily penalizing infrequent use of oversupply of power, as users get charged per kilowatt (kW) peak. Energy players, renewable developers, grid operators, and energy offtakers who take action soon could overcome these major roadblocks. There are various actions each one could take….Renewable developers and utilities could optimize their generation and client portfolios, combining generation and storage, and implement advanced analytics tools to improve production value — provided they carefully manage the increased merchant risk of having a relatively fixed cost and variable price set by the market”
All this is to say that the offtake and short-term markets remain illiquid and we believe this will persist well into the future without a significant shift in the market structure regarding price discovery and without the ability to tranche renewable assets for baseline load in aggregate. To illustrate the significance of this we point the reader to the following quote from a letter written from the EU General Secretariat to the Permanent Representatives Committee:
“Intraday markets are particularly important for the integration of variable renewable energy sources in the electricity system at the least cost as they give the possibility to market participants to trade shortages or surplus of electricity closer to the time of delivery. Since variable renewable energy generators are only able to accurately estimate their production close to the delivery time, it is crucial for them to have a maximum of trading opportunities via access to a liquid market as close as possible to the time of delivery of the electricity”
It’s important to note that the liquidity problem cannot be solved exclusively via the use of offtake contracts, marginal infrastructural changes or even storage for the reasons stated. A renewable energy price discovery mechanism based on a permissionless, collaborative “Nash Equilibrium” for tranched, baseline load that offers liquidity in both the long and short-term markets, can resolve this issue.
Policy-makers in North America and the EU may wish to consider this perspective and move towards the adoption of a market-based solution such as the use of Distributed Dynamic Hedge (DDH) technology. This technology is based on FinTech, DeFi and Blockchain principles of decentralization, permissionless participation and price-risk sharing. It would allow the renewables market to benefit from aggregate tranching while also being de-fragmented in order to create a true futures market for renewable energy based on a uniform price and forward supply that serves regional baseline demand. This would then enable much higher RPS quotas.
More on that in our next write-up…